Volatile analysis of the Hayausa2 asteroid Ryugu sample revealing volatile sources and recent surface evolution has been published in “Science”.
October 21, 2022 (JST)
Japan Aerospace Exploration Agency
Kyushu University
Ibaraki University
Hokkaido University
Tohoku University
Kyoto University
Hiroshima University
The University of Tokyo
The Japan Aerospace Exploration Agency (JAXA) is conducting the first analysis of the sample returned from asteroid Ryugu by the Hayabusa2 mission with the Hayabusa2 Initial Analysis Team, comprising of six sub-teams and two Phase-2 curation institutes. Results from the Initial Analysis Team’s “Volatile Component Analysis Team” have been published in the US Journal “Science” on October 21, 2022 (JST).
Title:Noble gases and nitrogen in samples of asteroid Ryugu record its volatile sources and recent surface evolution
The initial analysis of the asteroid Ryugu samples
The sample from asteroid Ryugu was returned to Earth by the Hayabusa2 spacecraft on December 6, 2020. The initial description of the sample (Phase-1 curation) followed as the facility established at JAXA’s Institute of Space and Astronautical Science (ISAS). Parts of the sample were then distributed to the Hayabusa2 Initial Analysis Team, which consists of six sub-teams and two Phase-2 curation institutes. This division into specialized sub-teams is designed to achieve the scientific objectives of Hayabusa2 through high-precision analysis of different aspects of the sample. Data from the Phase-2 curation institutes’ comprehensive analysis of the grain characteristics will be included in the JAXA Hayabusa2 grain catalogue for future analysis proposals. Reports from the six initial analysis teams and Phase-2 curation institutes are being announced individually as the results are published in scientific journals. After all the results of the initial analysis have been announced, an overall summary is planned.
[Appendix]
Noble gases and nitrogen in samples from asteroid Ryugu record
volatile sources and recent surface evolution
1. Overview
This analysis measured the isotopic composition of the noble gases and nitrogen in the surface and sub-surface material from the near-Earth asteroid Ryugu, which was returned to Earth by the Hayabusa2 mission. The abundance and isotopic compositions of volatile elements such as noble gases and nitrogen tells us about the original materials that formed the parent asteroid of Ryugu, fragments of which later accumulated to form the present-day Ryugu. Volatile abundances can be affected by processes in the asteroid, such as heating or reactions with water. In this research, noble gases and nitrogen in Ryugu were investigated as a probe for the origin and evolution of Ryugu from the beginning of the formation the parent asteroid to the current surface geological processes.
The Ryugu sample was found to contain primitive noble gas from the early Solar System in abundances greater than that found in any meteorite reported to date. The nitrogen isotope composition differs within the sample grains, indicating that a variety of nitrogen-baring materials from the early Solar System are still preserved within the Ryugu sample.
As well as the primordial gas from the formation of the early Solar System, the sample contained two additional types of noble gases, one produced by reactions triggered by the irradiation of galactic cosmic rays, and the other originating from the implantation of the solar wind. The abundance of solar wind-derived noble gases contained in many of the Ryugu grains was very small. Of the ten grains recovered from the first touchdown and the six grains recovered from the second touchdown that were analysed, most did not contain solar wind noble gases. Only two grains contained a clear signature of solar wind noble gases equivalent to 3,500 years and 250 years of irradiation respectively, at Ryugu’s present orbit. Since the solar wind only hits the outermost layer of the rocky material, these grains must have been on the outermost layer for 3,500 years and 250 years, respectively. The second touchdown sample was collected near the artificial crater and is expected to contain sub-surface material. Since this second touchdown material does not contain much solar wind-derived noble gases, it was concluded that the sub-surface material at a depth of 1-2m has not been stirred greatly.
The amount of galactic cosmic ray-produced neon suggests that the period of galactic cosmic ray irradiation of the Ryugu sample was about 5 million years. Two ages have been proposed for the surface of Ryugu, based on the number of craters. An age of 2 to 8 million years is estimated assuming that the craters were formed due to collisions while Ryugu was in a near-Earth orbit, while an age of 100,000 to 300,000 years is estimated for craters via more frequent impacts in the asteroid belt. The galactic cosmic ray irradiation period obtained from the noble gas analysis is consistent with the former age, suggesting that Ryugu moved from an orbit within the asteroid belt to a near-Earth orbit where there were fewer surface impacts about 5 million years in the past (Figure 1).
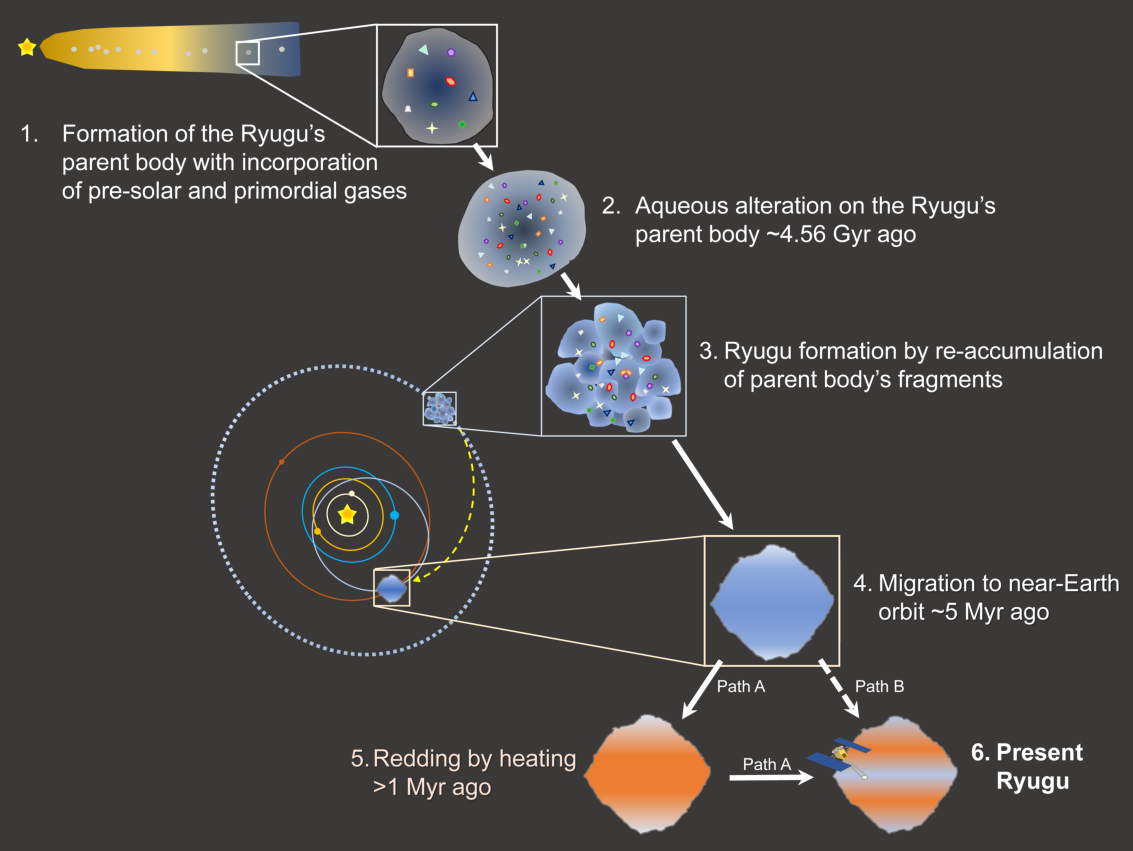
©Okazaki et al., 2022a
Figure 1: Diagram of the evolution of asteroid Ryugu.
When Ryugu sample grains were heated to a temperature of 100℃ in the vacuum chamber, part of the noble gases produced by galactic cosmic rays was released which corresponded to an irradiation period of 1 million years. This implies that the Ryugu surface material has not experienced temperatures of 100℃ during the past million years. Multi-band imaging from the Hayabusa2 spacecraft of the mid-latitude region of Ryugu revealed material that appeared reddish (reflected more strongly at redder wavelengths). Previous studies have suggested that this reddish material may have been formed when Ryugu was intensely heated due to being close to the Sun for a period of time. If the cause of this reddening is heating from proximity to the Sun, then it must have occurred more than a million years ago (Figure 1).
2.Text
In order to analyse the volatile components, the volatile sub-team created pellets from individual grains with sizes less than 1mm (0.3 mg or less in weight: total of 24 grains) to obtain a flat surface. This was done in an environment of nitrogen gas, without exposing the sample to the atmosphere. Infrared spectroscopic analysis and observations using a scanning electron microscope were then performed on the pellet surface. This revealed that the Ryugu sample allocated to the volatile sub-team have petrology and mineralogy that is similar to the Ivuna meteorite, which is a carbonaceous chondrite meteorite (CI chondrites), as has been found with other Ryugu samples. Destructive analysis to examine noble gas and nitrogen isotopes was then performed on these pelletized samples.
Noble gas isotope measurements were performed on ten sample grains that were collected during the first touchdown by the Hayabusa2 spacecraft (Ryugu-A sample: collection from the outermost layer of asteroid Ryugu), and six sample grains collected from near the artificial crater during the second touchdown (Ryugu-C sample: expected to contain sub-surface material ejected from the creation of the artificial crater). The Ryugu samples were found to contain a large amount of carbonaceous material and pre-Solar System material (such as nano-diamonds and graphite) that existed in the Solar System at the time of its formation. The isotopic composition is similar to that of CI chondrites; a type of carbonaceous chondrite that is considered to be the most primitive type of meteorite. Meanwhile, the concentration of noble gases contained within the Ryugu grains was higher than that in both CI chondrites and other types of chondrites (Figure 2). This indicates that the material that formed the parent body of Ryugu (from which the present-day Ryugu formed by re-accumulation of broken fragments) is composed of the same kinds of materials as the CI chondrites, but more enriched in carbonaceous material and pre-solar system material.
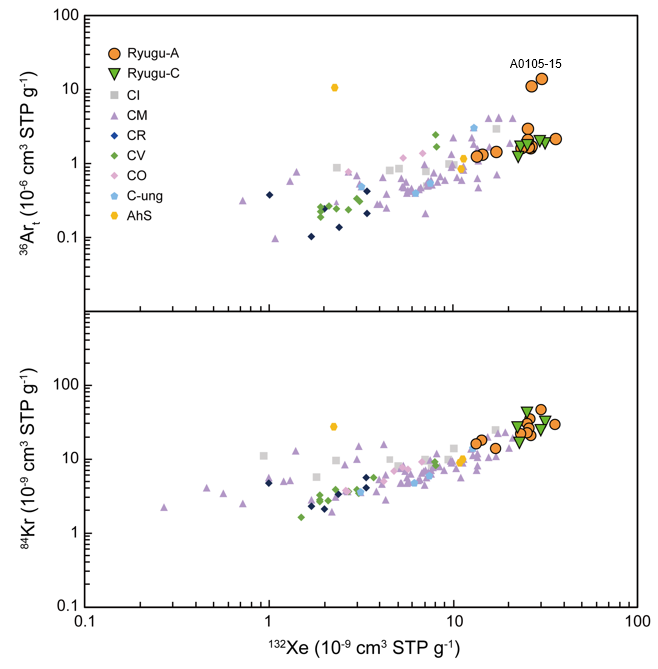
©Okazaki et al., 2022a
Figure 2: concentration of primordial noble gases in the Ryugu sample. The vertical axis shows concentrations of the primordial noble gases 36Ar and 84Kr, while the horizontal axis shows the 132Xe concentration. The grains from Ryugu (orange circles and green inverted triangles) contain higher concentrations of noble gases originating from the formation of the early Solar System than previously known meteorites. Carbonaceous chondrites (grey squares, purple triangles, light blue pentagons) and other meteorite material (orange hexagons) are also shown for reference.
From the analysis of four of the Ryugu sample grains (two from the Ryugu-A sample, and two from the Ryugu-C sample), the isotopic composition and concentration of nitrogen was found to vary between the Ryugu grains (Figure 3). This suggests that the variety of nitrogen-bearing components that formed Ryugu’s parent body in the early Solar System still remain on present-day Ryugu. Some of the Ryugu grains are deficient in the 15N isotope, suggesting that 15N-rich materials were lost during aqueous alteration and possibly dehydration processes that occurred within Ryugu’s parent body.
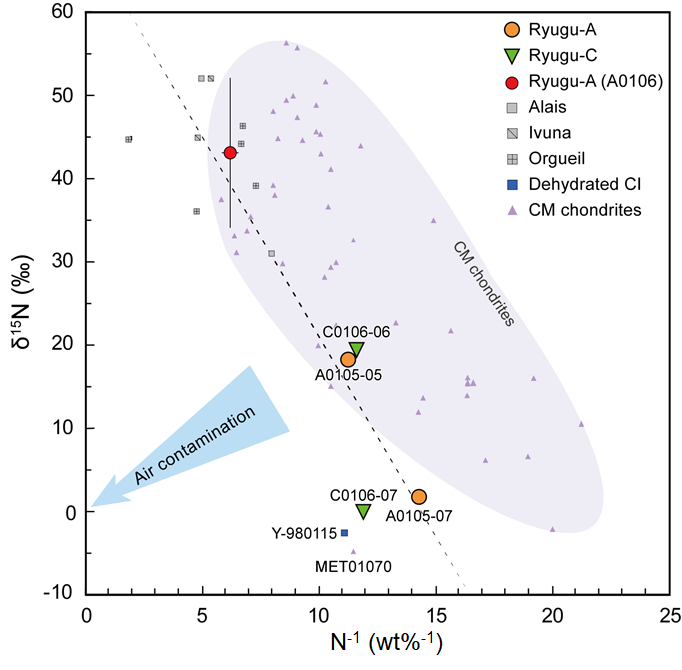
©Okazaki et al., 2022a
Figure 3: The Nitrogen isotope composition of the Ryugu sample grains (vertical axis, measured as difference from the Earth’s atmosphere in parts per thousand) vs the inverse nitrogen abundance (weight ratio: horizontal axis). The Ryugu grains (orange circles, green inverted triangles, red circle) each show a different nitrogen composition. CI chondrites (grey and blue squares) and CM chondrites (purple triangles, purple enclosed area) is also shown for reference. If mixed with the Earth’s atmosphere, the analysis data would move in the direction indicated by the blue arrow, but the effect would not be visible.
In addition to the primordial gas present in the early Solar System, the sample grains contained two types of noble gases: galactic cosmic rays-produced noble gases and implanted solar wind noble gases (figure 4). Ten Ryugu-A samples and six Ryugu-C sample grains were analysed, but only two of the Ryugu-A samples contained substantial quantities of helium and neon originating from the solar wind. The irradiation of the solar wind needed to produce the observed abundances of noble gasses in these two grains were calculated to be 3,500 years and 250 years respectively, assuming asteroid Ryugu is in its current orbit. This irradiation period is shorter than that of the grains from asteroid Itokawa (returned by the Hayabusa mission in 2010) and of lunar regolith particles. In addition, the frequency of the grains with a solar wind noble gases is extremely low in the Ryugu sample compared to Itokawa and lunar particles.
In other Ryugu grains, the amount of solar wind noble gases was found to be small, with an irradiation period calculated at less than several decades. Most of the material recovered from Ryugu’s surface could therefore only have been on the outermost surface for this short period of a few decades.
The sample grains containing a substantial amount of solar wind noble gas were found only in the Ryugu-A sample, with the sample retrieved from the second touchdown (presumed to contain sub-surface material) not containing much solar wind noble gas. Since the penetration depth of the solar wind is very small and the artificial crater has a depth of about 1.7m, this means that the sub-surface of asteroid Ryugu to a depth of about 1-2 m has not been mixed significantly.
The average quantity of neon produced by galactic cosmic rays was also calculated for the Ryugu-A (ten grains) and Ryugu-C (six grains) sample. Based on production rates of cosmic ray-produced neon that were theoretically calculated assuming the cosmic ray irradiation for material 2 - 4 cm just below the surface (for Ryugu-A grains) and down to about 1.3m (for Ryugu-C grains that were likely ejected from the artificial crater at around this depth), results from both samples were found to be consistent with Ryugu being exposed to cosmic rays for about 5 million years.
Both this result and that from the solar wind noble gas abundance suggests that the region 1-2 m below the surface of Ryugu has not been frequently agitated and mixed by meteorite impact. This is consistent with a low impact frequency of meteorites on the Ryugu surface which is expected in the current near-Earth orbit of asteroid Ryugu. Crater counts combined with this expected impact frequency suggest a surface age of 2 to 8 million years. The other estimate of Ryugu’s crater age based on a more frequent impact rate that would be found in an orbit within the asteroid belt is 100,000 to 300,000 years old, but does not agree with this analysis result which points to an infrequent meteorite impact rate for the past 5 million years. It is therefore thought that Ryugu moved from the asteroid belt to its current near-Earth orbit about 5 million years ago (Figure 1).
It was also found that the neon produced by galactic cosmic ray irradiation and the solar wind neon can be liberated simply by heating the sample to 100℃. From the amount of released galactic cosmic ray-produced neon, Ryugu’s surface material cannot have been heated about 100℃ during the past one million years. Other studies that have also estimated the temperature experienced by surface materials are consistent with this finding.
This study was able to also obtain an age estimate of surface processes, in addition to the temperature information. The mid-latitude regions on the surface of asteroid Ryugu appeared reddish in the multi-band visible imaging performed by the telescope camera on the Hayabusa2 spacecraft. Previous studies based on this on-site analysis have argued that the reddish material may have been formed during strong sunlight heating of Ryugu during a period of time when the asteroid was close to the Sun. If this cause of reddening is indeed due to heating, then this occurred more than one million years in the past (Figure 1).
Hayabusa2 created an artificial crater on the asteroid surface in a world’s first, and as a result, was able to collect sub-surface material from asteroid Ryugu. By comparing the solar wind-derived noble gases in the asteroid’s outermost layer material and in the sub-surface material, we found for the first time that the evolutionary history of the surface and sub-surface materials are different.
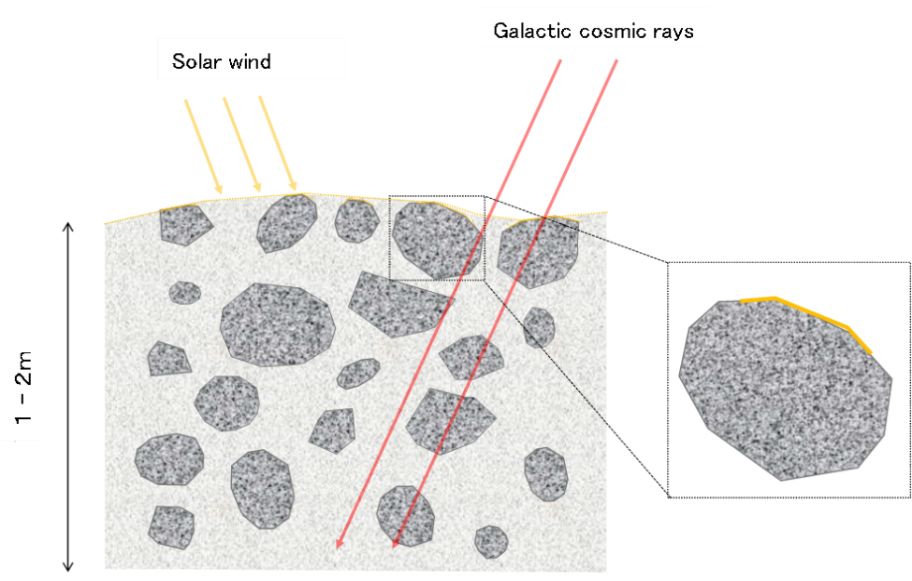
©Kyushu University
Figure 4: Solar wind (orange arrows) and the galactic cosmic ray irradiation (red arrows) on the asteroid surface. The solar wind reaches only 100 nm into the surface material, while galactic cosmic rays can affect depths down to 1-2 m.
3. Publication information
Journal Title: Science
DOI: 10.1126/science.abo0431
Title of Paper:Noble gases and nitrogen in samples of asteroid Ryugu record its volatile sources and recent surface evolution
Authors:R. Okazaki1, B. Marty2, H. Busemann33, K. Hashizume4, J.D. Gilmour5, A. Meshik6, T. Yada7, F. Kitajima1, M.W. Broadley2, D. Byrne2, E. Füri2, M.E.I. Riebe3, D. Krietsch3, C. Maden3, A. Ishida8, P. Clay5, S.A. Crowther5, L. Fawcett5, T. Lawton5, O. Pravdivtseva6, Y.N. Miura9, J. Park10,11, K. Bajo12, Y. Takano13, K. Yamada14, S. Kawagucci15,16, Y. Matsui15,16, M. Yamamoto1, K. Righter17, S. Sakai13, N. Iwata18, N. Shirai19,20, S. Sekimoto21, M. Inagaki21, M. Ebihara19, R. Yokochi22, K. Nishiizumi23, K. Nagao24, J.I. Lee24, A. Kano25, M.W. Caffee26,27, R. Uemura28, T. Nakamura8, H. Naraoka1, T. Noguchi29, H. Yabuta30, H. Yurimoto12, S. Tachibana31, H. Sawada7, K. Sakamoto7, M. Abe7,32, M. Arakawa33, A. Fujii7, M. Hayakawa7, N. Hirata33, N. Hirata34, R. Honda35, C. Honda34, S. Hosoda7, Y. Iijima7†, H. Ikeda7, M. Ishiguro36, Y. Ishihara37, T. Iwata7, K. Kawahara7, S. Kikuchi38,39, K. Kitazato34, K. Matsumoto32,39, M. Matsuoka40, T. Michikami41, Y. Mimasu7, A. Miura7, T. Morota25, S. Nakazawa7, N. Namiki32,39, H. Noda32,39, R. Noguchi42, N. Ogawa7, K. Ogawa37, T. Okada7,43, C. Okamoto33†, G. Ono44, M. Ozaki7,32, T. Saiki7,32, N. Sakatani45, H. Senshu38, Y. Shimaki7, K. Shirai7,33, S. Sugita25, Y. Takei7, H. Takeuchi7, S. Tanaka7, E. Tatsumi25,46, F. Terui47, R. Tsukizaki7, K. Wada38, M. Yamada38, T. Yamada7, Y. Yamamoto7, H. Yano7,32, Y. Yokota7, K. Yoshihara7, M. Yoshikawa7,32, K. Yoshikawa7, S. Furuya7, K. Hatakeda48, T. Hayashi7, Y. Hitomi48, K. Kumagai48, A. Miyazaki7, A. Nakato7, M. Nishimura7, H. Soejima48, A. Iwamae48, D. Yamamoto7,49, K. Yogata7, M. Yoshitake7, R. Fukai7, T. Usui7, H.C. Connolly Jr.50, D. Lauretta51, S. Watanabe28, Y. Tsuda7
1Department of Earth and Planetary Sciences, Kyushu University, Fukuoka, 819-0395, Japan.
2Université de Lorraine, CNRS, CRPG, F-54000 Nancy, France.
3Institute of Geochemistry and Petrology, Eidgenössische Technische Hochschule (ETH) Zürich, 8092 Zürich, Switzerland.
4Faculty of Science, Ibaraki University, Mito, 310-8512, Japan.
5Department of Earth and Environmental Sciences, The University of Manchester, Manchester M13 9PL, UK.
6Physics Department, Washington University, Saint Louis, MO, 63130, USA.
7Institute of Space and Astronautical Science, Japan Aerospace Exploration Agency (JAXA), Sagamihara, 252-5210, Japan.
8Department of Earth Science, Tohoku University, Sendai, 980-8578, Japan.
9Earthquake Research Institute, The University of Tokyo, Tokyo, 113-0032, Japan.
10Physical Sciences, Kingsborough Community College, The City University of New York, Brooklyn, NY, 11235, USA.
11Department of Earth and Planetary Sciences, American Museum of Natural History, NY, 10024, USA.
12Department of Earth and Planetary Sciences, Hokkaido University, Sapporo, 060-0810, Japan.
13Biogeochemistry Research Center, Japan Agency for Marine-Earth Science and Technology (JAMSTEC), Yokosuka, 237-0061, Japan.
14Department of Chemical Science and Engineering, Tokyo Institute of Technology, Yokohama, 226-8503, Japan.
15Research Institute for Global Change, JAMSTEC, Yokosuka, 237-0061, Japan.
16Institute for Extra-cutting-edge Science and Technology Avant-garde Research (X-star), JAMSTEC, Yokosuka, 237-0061, Japan.
17Astromaterials Research and Exploration Science, Mailcode XI2, National Aeronautics and Space Administration (NASA) Johnson Space Center, Houston, TX, 77058, USA.
18Faculty of Science, Yamagata University, Yamagata, 990-8560, Japan.
19Graduate School of Science and Engineering, Tokyo Metropolitan University, Hachioji, 192-0397, Japan.
20Department of Chemistry, Faculty of Science, Kanagawa University, Hiratsuka, Kanagawa, 259-1293, Japan.
21Institute for Integrated Radiation and Nuclear Science, Kyoto University, Osaka, 590-0494, Japan.
22Department of the Geophysical Sciences, The University of Chicago, Chicago IL, USA.
23Space Sciences Laboratory, University of California, Berkeley, CA, 94720, USA.
24Division of Earth Sciences, Korea Polar Research Institute, Incheon, 21990, Korea.
25School of Science, The University of Tokyo, Tokyo, 113-0033, Japan.
26Department of Physics and Astronomy, Purdue University, West Lafayette, IN 47907, USA.
27Department of Earth, Atmospheric, and Planetary Sciences, Purdue University, West Lafayette, IN 47907, USA.
28Department of Earth and Environmental Sciences, Nagoya University, Nagoya, 464-8601, Japan.
29Division of Earth and Planetary Sciences, Kyoto University, Kyoto, 606-8502, Japan.
30Department of Earth and Planetary Systems Science, Hiroshima University, Higashi-Hiroshima, 739-8526, Japan.
31UTokyo Organization for Planetary and Space Science, The University of Tokyo, Tokyo, 113-0033, Japan.
32Department of Space and Astronautical Science, The Graduate University for Advanced Studies, Hayama 240-0193, Japan.
33Department of Planetology, Kobe University, Kobe, 657-8501, Japan.
34Aizu Research Cluster for Space Science, University of Aizu, Aizu-Wakamatsu, 965-8580, Japan.
35Center of Data Science, Ehime University, Matsuyama, 790-8577, Japan.
36Department of Physics and Astronomy, Seoul National University, Seoul, 08826, Republic of Korea.
37JAXA Space Exploration Center, JAXA, Sagamihara, 252-5210, Japan.
38Planetary Exploration Research Center, Chiba Institute of Technology, Narashino, 275-0016, Japan.
39National Astronomical Observatory of Japan, Mitaka, 181-8588, Japan.
40Geological Survey of Japan, National Institute of Advanced Industrial Science and Technology, Ibaraki, 305-8567, Japan.
41Faculty of Engineering, Kindai University, Higashi-Hiroshima, 739-2116, Japan.
42Faculty of Science, Niigata University, Niigata, 950-2181, Japan.
43Department of Chemistry, The University of Tokyo, Tokyo 113-0033, Japan.
44Research and Development Directorate, JAXA, Sagamihara, 252-5210, Japan.
45Department of Physics, Rikkyo University, Tokyo, 171-8501, Japan.
46Instituto de Astrofísica de Canarias, University of La Laguna, Tenerife, Spain.
47Department of Mechanical Engineering, Kanagawa Institute of Technology, Atsugi, 243-0292, Japan.
48Marine Works Japan Ltd., Yokosuka, 237-0063, Japan.
49Department of Earth and Planetary Science, Tokyo Institute of Technology, Ookayama, Tokyo, 152-8550, Japan.
50Department of Geology, School of Earth and Environment, Rowan University, Glassboro, NJ, 08028, USA.
51Lunar and Planetary Laboratory, University of Arizona, Tucson, AZ, 85705, USA.
†Deceased.