Asteroid Explorer Hayabusa2 Initial Analysis: Soluble Organic Matter (SOM) Team reveals organic molecule compositions in samples of asteroid Ryugu
February 24, 2023 (JST)
Japan Aerospace Exploration Agency
Kyushu University
Japan Agency for Marine-Earth Science and Technology
Nagoya University
Kyoto University
Hiroshima University
Tohoku University
Hokkaido University
The University of Tokyo
The Japan Aerospace Exploration Agency (JAXA) is conducting the first analysis of the sample returned from asteroid Ryugu by the Hayabusa2 mission with the Hayabusa2 Initial Analysis Team, comprising of six sub-teams and two Phase-2 curation institutes. Results from the Initial Analysis Team’s “Soluble Organic Matter (SOM) Team” have been published in the American scientific Journal “Science”, on February 24, 2023 (JST).
Paper Title: | Soluble organic molecules in samples of the carbonaceous asteroid (162173) Ryugu |
---|---|
Journal: | Science |
DOI: | |
Please refer to the Appendix for an outline. |
The Initial analysis of the asteroid Ryugu samples
The sample from asteroid Ryugu returned to Earth by the asteroid explorer, Hayabusa2, on December 6, 2020, initially underwent a cataloguing description (Phase-1 curation) at the facility established at JAXA’s Institute of Space and Astronautical Science. Part of the returned sample was distributed to the Hayabusa2 Initial Analysis Team, consisting of six sub-teams, and two Phase-2 curation institutes at Okayama University and JAMSTEC Kochi Institute for Core Sample Research. The initial analysis is designed to reveal the multifaceted features of the sample through a plan of high-precision analysis, with specialized sub-teams assigned to tackle the science objectives of the Hayabusa2 mission. Meanwhile, the Phase-2 curation institutes have specific specialties that are utilized to catalogue the sample based on a comprehensive analysis flow, and clarify the potential impact of the sample through measurement and analysis appropriate to the characteristics of the returned particles.
Appendix
Soluble organic molecules in samples of the carbonaceous asteroid (162173) Ryugu
1.Key points
● | The solvent extractable organic molecules were analyzed by ultra-high resolution mass spectrometry, and found to contain approximately 20,000 organic molecules composed of carbon (C) and hydrogen (H), nitrogen (N), oxygen (O), and/or sulfur (S). |
---|---|
● | Using gas and liquid chromatography, amino acids, carboxylic acids, amines, and aromatic hydrocarbons were detected. In particular, the presence of small, highly volatile organic molecules such as methylamine and acetic acid indicates that these molecules are stable as salts on the Ryugu surface. |
● | Both proteinogenic amino acids (such as alanine) and non-proteinogenic amino acids (such as isovaline) were found. Enantiomeric amino acids having left and right structures were present at approximately 1:1 as racemic mixtures. Hence, the detection of these racemic amino acids are important evidence of abiotic origin. |
● | Alkylbenzenes and polycyclic aromatic hydrocarbons such as naphthalene, phenanthrene, pyrene, and fluoranthene were the main hydrocarbons. The pattern of their occurrence is similar to that of hydrothermal crude oils on Earth, suggesting that they were influenced by aqueous alteration on the Ryugu parent body. |
● | In situ analysis of the sample surface sprayed with methanol showed that different organic molecules were present in different spatial distributions, suggesting that the organic compounds may have migrated and separated during the interaction of fluids and minerals on the Ryugu parent body. |
● | It has been observed that materials are ejected into space from asteroid surfaces by various processes, and organic molecules from Ryugu's surface could be transported to other celestial bodies. |
2.Outline
Soluble organic molecules in the sample of asteroid Ryugu brought back by Hayabusa2 were analyzed mainly by sequential extraction using hydrophobic to hydrophilic solvents. Ryugu is a dark primitive asteroid classified into the C-type asteroid, which is the most common in the asteroid belt, and is rich in hydrous minerals such as carbonaceous chondrite meteorites. Primitive carbonaceous chondrites are known to contain a variety of soluble organic molecules, including amino acids, and might have supplied prebiotic organic molecules to the primitive Earth and other celestial bodies. In this study, organic molecules in Ryugu surface samples obtained from the first touchdown sampling site were analyzed by the international research team from Japan, the U.S., and Europe. Wide variety of organic molecules such as amino acids, amines, carboxylic acids, aromatic hydrocarbons, and nitrogen-containing cyclic compounds were detected (Figure 1). These organic molecules may be released from Ryugu's surface and transported to other celestial bodies in the Solar system.
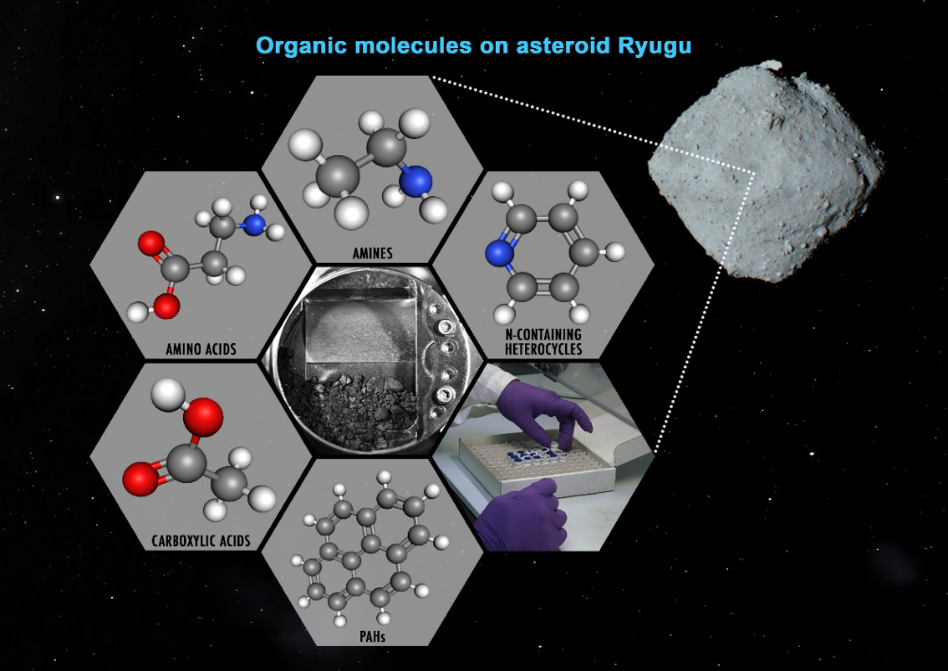
©JAXA, University of Tokyo, Kochi University, Rikkyo University, Nagoya University, Chiba Institute of Technology, Meiji University, University of Aizu, AIST, NASA, Dan Gallagher.
Figure 1. Conceptual organic molecules found in surface samples from the asteroid Ryugu
3.Main text
The main analyses and results performed by the Soluble Organic Matter (SOM) Team of the Hayabusa2 Sample Initial Analysis Team are as follows.
First, elemental analyses and isotopic ratio measurements were performed for carbon (C) and nitrogen (N), hydrogen (H), sulfur (S) and pyrolytic oxygen (O) to evaluate the average of Ryugu regolith composition. The Ryugu aggregate sample (A0106) contained about 3.8% C, 1.1% H, 0.16% N, 3.3% S, and 12.9% pyrolytic O. The total of these volatile light elements CHNOS was about 21.3%. These elements constitute inorganic minerals such as hydrous silicates, carbonates, and sulfides, as well as soluble and insoluble organic matter. To date, these elemental abundances are the highest profiles among the previous descriptions of carbonaceous meteorites, indicating that Ryugu is rich in volatile elements (Figure 2). The stable isotopic composition (Note 1) of carbon (13C/12C)δ13C is about -0.6 permil, nitrogen (15 N/14N), δ15N is about +43 permil, hydrogen (D/H), δD is about +250 permil, sulfur (34S/32S)δ34S is about -3 permil, and the closest comparison to the meteorite is the CI (Ivuna type) carbonaceous chondrite meteorite.
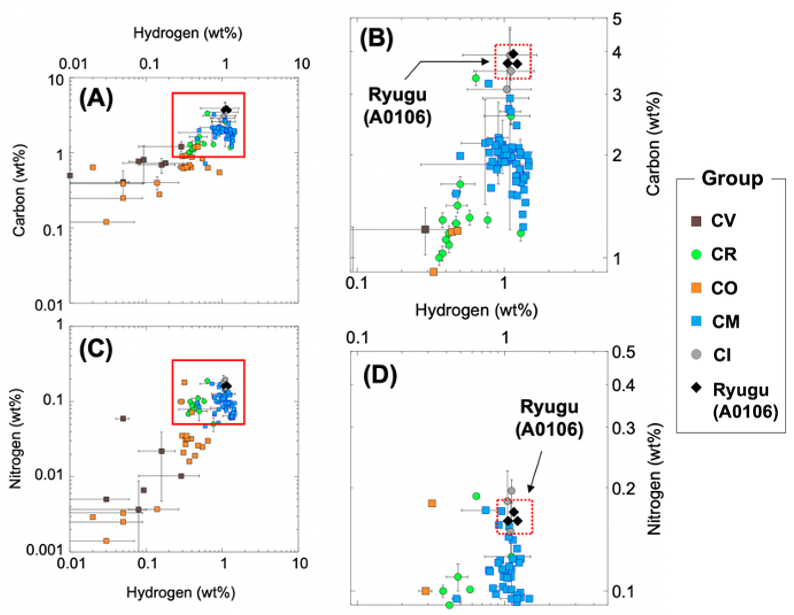
©H. Naraoka et al. Science 379, eabn9033 (2023)
Figure 2. Carbon, hydrogen, and nitrogen content of asteroid Ryugu samples
The methanol extract of the Ryugu sample was analyzed using electrospray ionization (ESI) (Note 2) and atmospheric pressure photoionization (APPI) (Note 3) coupled with a Fourier transform-ion cyclotron resonance mass spectrometer (FT-ICR/MS) (Note 4), which revealed about 20,000 organic molecular ions up to about 700 Da consisting of CH, CHO, CHN, CHS, CHNO, CHOS, CH, CHO, CHN, CHS, CHNO, CHOS, and CHNOS (Figure 3). Many of these constitute a series of homologous compounds, indicating a series of chemical reactions of organic molecules, such as methylation and hydroxylation. The diversity of these organic molecules is greater than that of carbonaceous meteorites, suggesting that they formed in a relatively low-temperature environment.
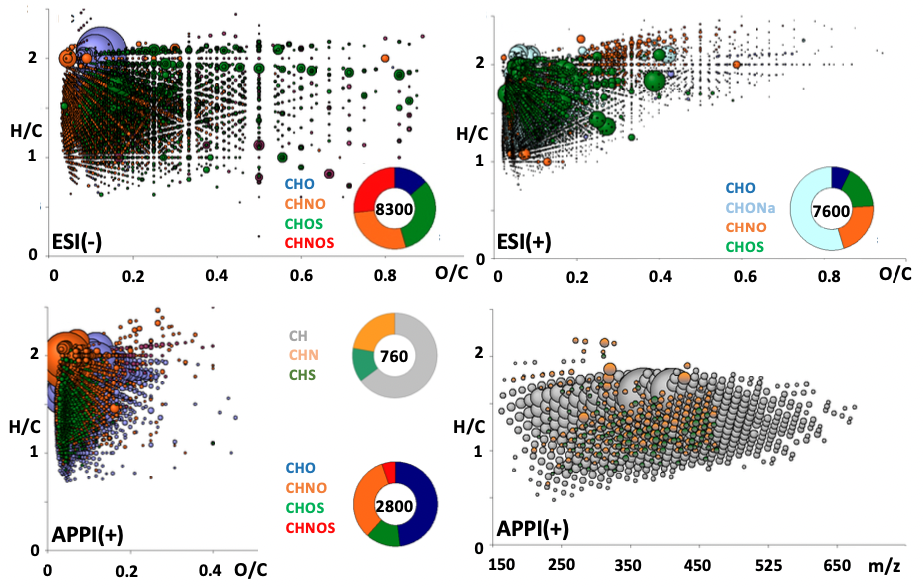
©H. Naraoka et al. Science 379, eabn9033 (2023)
Figure 3. Chemical composition of about 20,000 species detected in alcohol extracts of asteroid Ryugu samples.
Hot water extracted and acid-hydrolyzed fractions from the Ryugu sample were subjected to amino acid analysis by two independent analytical methods. Firstly, liquid chromatography fluorescence detection/high resolution mass spectrometry (LC-FD/HRMS) was used for total D,L-amino acid analysis. Secondly, 3D-high performance liquid chromatography with fluorescence detection (3D-HPLC/FD) was used for sensitive analysis specialized for separation of optical isomers of D,L-amino acids. As a result, 15 types of amino acids were detected, including proteinogenic amino acids such as α-alanine, glycine, and valine, which are common molecules in terrestrial life, and non-proteinogenic amino acids such as β-alanine, aminobutyric acid, and isovaline. The detected optical isomers (Note 5) were racemic, existing almost 1:1 ratios (Figure 4). Non-proteinogenic amino acids and their presence in racemic form indicates that they were synthesized in the prebiotic molecular evolution on Ryugu. The amino acid concentrations were typically less than 1 nanomole/g and as low as a few ppb and ultra-trace amounts. Much less than the Murchison meteorite, where a comparative wide variety of amino acids were detected, and less than the CI chondrite meteorite (Orgueil meteorite).
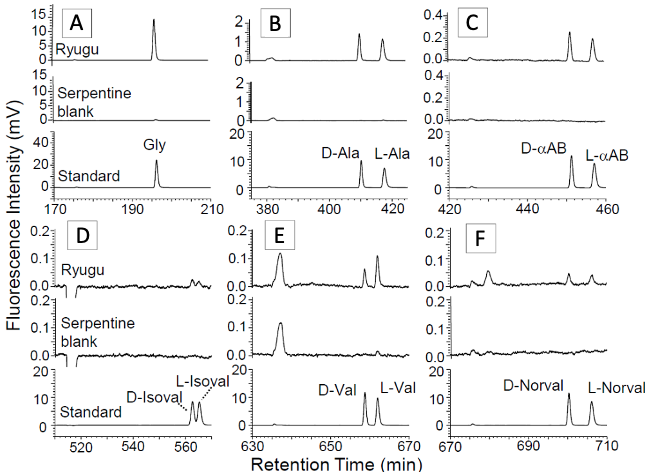
©H. Naraoka et al. Science 379, eabn9033 (2023)
Figure 4. Enantiomeric amino acids detected in hot water extracted and hydrolyzed fraction of the Ryugu sample.
In addition to amino acids, low molecular weight amines such as methylamine and carboxylic acids such as acetic acid were present in the hot water extract. For example, the boiling point of the free form of methylamine is -6.3℃ at 1 atm (1013 hPa), which is very volatile. On the Ryugu surface, it is shown to exist as a salt (e.g., the boiling point of methylamine hydrochloride is about 230℃ at 20 hPa). In addition to methylamine, ethylamine and propylamine were also present, but isopropylamine (branched) was more abundant than straight-chain propylamine. This indicates that they were either formed by radical reactions or were present after thermal decomposition. Butylamine, which is present in the Orgueil meteorite, was not detected. These amine salts may be related to the absorption band ~3.1µm (NH bonding) observed on the Ryugu surface. Acetic acid and formic acid were also present as carboxylic acids, but the higher carboxylic acids were not detected. The finding of only smaller carboxylic acids is consistent with the characteristics found in carbonaceous meteorites that have undergone hydrothermal alteration.
Aromatic hydrocarbons were detected in the fractions extracted with organic solvents. Alkylbenzenes and other alkyl chains to polycyclic aromatic hydrocarbons (PAHs) ranging from 2-ring naphthalene to 4-ring pyrene and fluoranthene, and their alkylated forms were detected. PAHs are thought to be ubiquitous in interstellar space, and are also abundant in carbonaceous meteorites. In particular, pyrene and fluoranthene are structural isomers with the same chemical formula (C16H10) and are present at nearly 1:1 in many carbonaceous meteorites such as Murchison. However, pyrene was more abundant than fluoranthene in the Ryugu sample. This peculiar PAH distribution was also found in CI-type Ivuna carbonaceous meteorites, and it is possible that the PAHs were separated during water-rock mineral interaction (i.e., asteroidal chromatography) due to differences in solubility within the Ryugu parent body. The presence of alkylbenzenes and an excess of pyrene relative to fluoranthene have also been found in hydrothermal crude oils on Earth. Hence, indigenous hydrothermal crude oil-like organic matter may exist on Ryugu.
Nitrogen-containing cyclic compounds were present as a series of extensively alkylated (-CH2 -) homologues, which may have been synthesized on the parent body from formaldehyde and ammonia. Nitrogen-containing cyclic compounds are commonly detected in carbonaceous meteorites, but the carbon number distribution of the alkylpyridine homologues (CnH2n-4N+) found in Ryugu differs from that of the well-studied Murchison meteorite. While the Murchison meteorite had an abundance distribution of C8 to C16 with a maximum of C11, the Ryugu meteorite had a distribution of C11 to C22 carbons with a maximum of C17. This indicates that the synthesis of organic molecules (elongation of carbon bonds) is affected by thermal and aqueous alteration history. The distribution of organic molecules was also examined by methanol-spraying the surface of approximately 1 mm-sized particles of the Ryugu sample (A0080) for the imaging mass spectrometry. These nitrogen-containing cyclic compounds were found to have different spatial distributions on the micrometer scale in the particles, depending on the carbon number and different chemical compositions (Figure 5). Such differences in the spatial heterogeneity of organic molecules may be due to the interaction among water, organic molecules, and minerals on the Ryugu.
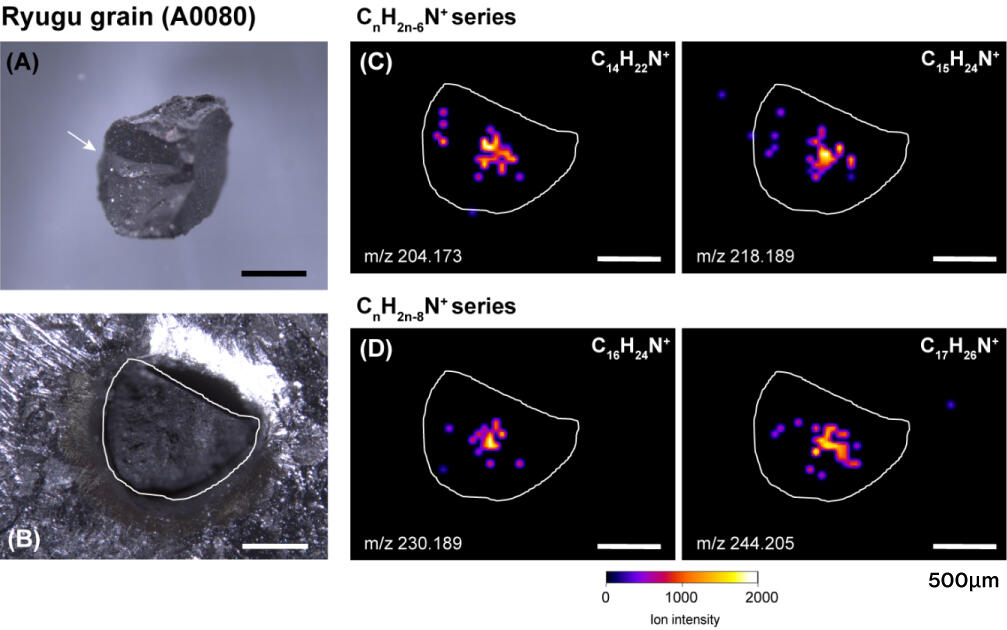
©H. Naraoka et al. Science 379, eabn9033 (2023)
Figure 5. Different spatial distributions of CHN compounds on the surface of Ryugu sample obtained by desorption electrospray ionization mass spectrometry.
The diversity of soluble organic molecules detected in the Ryugu samples is comparable to the diversity of organic molecules found in carbonaceous meteorites. However, the molecular distribution of low-molecular-weight organic compounds is relatively low, similar to the distribution of organic matter in carbonaceous meteorites that have been strongly affected by aqueous alteration. This is also consistent with mineralogical characteristics revealed by the initial analysis teams.
Asteroid surfaces are exposed to solar heating, ultraviolet, and high-energy cosmic rays under a high vacuum condition. However, this study shows that organic molecules are present on the regolith surface, probably protected by the shielding effect of minerals. Collisions and perturbations would eject organic material from the surface of carbonaceous asteroids, which would be transported to other bodies in the solar system as meteorites or cosmic dusts.
4.Paper information
Paper title:Soluble organic molecules in samples of the carbonaceous asteroid (162173) Ryugu
Authors:Naraoka, H.1, Takano, Y.2, Dworkin, J.P.3, Oba, Y.4, Hamase, K.5, Furusho, A., Ogawa, N.O.2, Hashiguchi, M.6, Fukushima, K.7, Aoki, D.7, Schmitt-Kopplin, P.8,9,10, Aponte, J.C.3, Parker, E.T.3, Glavin, D.P.3, McLain, H.L.3,11,12, Elsila, J.E.3, Graham, H.V.3, Eiler, J.M.13, Orthous-Daunay, F.-R.14, Wolters, C.14, Isa, J.15,16, Vuitton, V.14, Thissen, R.17, Sakai, S.2, Yoshimura, T.2, Koga, T.2, Ohkouchi, N.2, Chikaraishi, Y.4, Sugahara, H.18, Mita, H.19, Furukawa, Y.20, Hertkorn, N.8, Ruf, A.21,22,23, Yurimoto, H.24, Nakamura, T.20, Noguchi, T.25, Okazaki, R.1, Yabuta, H.26, Sakamoto, K., Tachibana, S.18,27, Connolly, Jr., H.C.28, Lauretta, D.S.29, Abe, M.18,30, Yada, T.18, Nishimura, M.18, Yogata, K.18, Nakato, A.18, Yoshitake, M.18, Suzuki, A.31, Miyazaki, A.18, Furuya, S.27, Hatakeda, K.31, Soejima, H.31, Hitomi, Y.31, Kumagai, K.31, Usui, T.18, Hayashi, T.18, Yamamoto, D.18, Fukai, R.18, Kitazato, K.32, Sugita, S.16,27, Namiki, N.30,33, Arakawa, M.34, Ikeda, H.18, Ishiguro, M.35, Hirata, N.31, Wada, K.16, Ishihara, Y.36, Noguchi, R.37, Morota, T.27, Sakatani, N.38, Matsumoto, K.38, Senshu, H.16, Honda, R.39, Tatsumi, E.40, Yokota, Y.18, Honda, C.32, Michikami, T.41, Matsuoka, M.18, Miura, A.18, Noda, H.30,33, Yamada, T.18, Yoshihara, K.18, Kawahara, K.18, Ozaki, M.18,30, Iijima, Y.18*, Yano, H.18,30, Hayakawa, M.18, Iwata, T.18, Tsukizaki, R.18, Sawada, H.18, Hosoda, S.18, Ogawa, K.42, Okamoto, C.34*, Hirata, N.33, Shirai, K.33, Shimaki, Y.18, Yamada, M.16, Okada, T.18,43, Yamamoto, Y.18,30, Takeuchi, H.18,30, Fujii, A.18, Takei, Y.18, Yoshikawa, K.36, Mimasu, Y.18, Ono, G.36, Ogawa, N.18, Kikuchi, S.16,33, Nakazawa, S.18, Terui, F.44, Tanaka, S.18,30, Saiki, T.18, Yoshikawa, M.18,30, Watanabe, S.6 and Tsuda, Y.18
- 1Department of Earth and Planetary Sciences, Kyushu University, Fukuoka 819-0395, Japan.
- 2Biogeochemistry Research Center, Japan Agency for Marine-Earth Science and Technology, Yokosuka 237-0061, Japan.
- 3Solar System Exploration Division, NASA Goddard Space Flight Center, Greenbelt, MD 20771, USA.
- 4Institute of Low Temperature Sciences, Hokkaido University, Sapporo 060-0189, Japan.
- 5Graduate School of Pharmaceutical Sciences, Kyushu University, Fukuoka 812-8582, Japan.
- 6Graduate School of Environment Studies, Nagoya University, Nagoya 464-8601, Japan.
- 7Graduate School of Bioagricultural Sciences, Nagoya University, Nagoya 464-8601, Japan.
- 8Helmholtz Munich, Analytical BioGeoChemistry, Neuherberg 85764, Germany.
- 9Technische Universität München, Analytische Lebensmittel Chemie, Freising 85354, Germany.
- 10Max Planck Institute for Extraterrestrial Physics, Garching bei München 85748, Germany.
- 11Center for Research and Exploration in Space Science and Technology, NASA Goddard Space Flight Center, Greenbelt, MD 20771, USA.
- 12Department of Physics, The Catholic University of America, Washington, D.C. 20064, USA.
- 13Division of Geological and Planetary Sciences, California Institute of Technology, Pasadena, CA 91125, USA.
- 14Université Grenoble Alpes, Centre National de la Recherche Scientifique (CNRS), Centre National d'Etudes Spatiales, L'Institut de Planétologie et d'Astrophysique de Grenoble, Grenoble 38000, France.
- 15Earth-Life Science Institute, Tokyo Institute of Technology, Tokyo 152-8550, Japan.
- 16Planetary Exploration Research Center, Chiba Institute of Technology, Narashino 275-0016, Japan.
- 17Université Paris-Saclay, CNRS, Institut de Chimie Physique, Orsay 91405, France.
- 18Institute of Space and Astronautical Science, Japan Aerospace Exploration Agency (JAXA), Sagamihara 252-5210, Japan.
- 19Department of Life, Environment and Material Science, Fukuoka Institute of Technology, Fukuoka 811-0295, Japan.
- 20Department of Earth Science, Tohoku University, Sendai 980-8578, Japan.
- 21Université Aix-Marseille, CNRS, Laboratoire de Physique des Interactions Ioniques et Moléculaires, Marseille 13397, France.
- 22Department of Chemistry and Pharmacy, Ludwig-Maximilians-University, Munich 81377, Germany.
- 23Excellence Cluster ORIGINS, Garching 85748, Germany.
- 24Department of Earth and Planetary Sciences, Hokkaido University, Sapporo 060-0810, Japan.
- 25Division of Earth and Planetary Sciences, Kyoto University, Kyoto 606-8502, Japan.
- 26Department of Earth and Planetary Systems Science, Hiroshima University, Higashi-Hiroshima 739-8526, Japan.
- 27Department of Earth and Planetary Science, University of Tokyo, Tokyo 113-0033, Japan.
- 28Department of Geology, School of Earth and Environment, Rowan University, Glassboro, NJ 08028, USA.
- 29Lunar and Planetary Laboratory, University of Arizona, Tucson, AZ 85721, USA.
- 30School of Physical Sciences, The Graduate University for Advanced Studies, Hayama 240-0193, Japan.
- 31Marine Works Japan Ltd., Yokosuka 237-0063, Japan.
- 32Aizu Research Cluster for Space Science, University of Aizu, Aizu-Wakamatsu 965-8580, Japan.
- 33Research of Interior Structure and Evolution of Solar System Bodies, National Astronomical Observatory of Japan, Mitaka 181-8588, Japan.
- 34Department of Planetology, Kobe University, Kobe 657-8501, Japan.
- 35Department of Physics and Astronomy, Seoul National University, Seoul 08826, Republic of Korea.
- 36Research and Development Directorate, JAXA, Sagamihara 252-5210, Japan.
- 37Faculty of Science, Niigata University, Niigata 950-2181, Japan.
- 38Department of Physics, Rikkyo University, Tokyo 171-8501, Japan.
- 39Center of Data Science, Ehime University, Matsuyama 790-8577, Japan.
- 40Instituto de Astrofísica de Canarias, University of La Laguna, Tenerife E-38205, Spain.
- 41Faculty of Engineering, Kindai University, Higashi-Hiroshima 739-2116, Japan.
- 42JAXA Space Exploration Center, JAXA, Sagamihara 252-5210, Japan.
- 43Department of Chemistry, University of Tokyo, Tokyo 113-0033, Japan.
- 44Department of Mechanical Engineering, Kanagawa Institute of Technology, Atsugi 243-0292, Japan.
- *Deceased.
Journal: Science
5.Notes
-
(Note 1) Stable isotopes
Elements such as carbon (C), nitrogen (N), hydrogen (H), oxygen (O), and sulfur (S) have a fixed weight (mass number). The same element with a different mass number is called an isotope, and those that exist stably (not radiogenic) are called stable isotopes. Stable isotope ratios of extraterrestrial materials may differ from those of terrestrial materials. -
(Note 2) Electrospray ionization (ESI)
This is one of the ionization methods used in mass spectrometry with solution samples. Since individual organic molecules can be ionized without fragments, primary information on the molecule (parent ion) can be obtained. It has excellent evaluability for groups of molecules that contain many polar functional groups. -
(Note 3) Atmospheric pressure photoionization (APPI)
Unlike ESI described above, this method uses ultraviolet light to ionize an introduced solution sample, making it possible to ionize nonpolar molecules such as hydrocarbons that are difficult to ionize with ESI. -
(Note 4) Fourier transform-ion cyclotron resonance mass spectrometer (FT-ICR/MS)
A Fourier transform mass spectrometer using a superconducting magnet that can determine precise ion masses to 5-6 decimal places with ultra-high mass resolution, and obtain comprehensive molecular group data with high sensitivity and resolution. -
(Note 5) Optical isomers
Compounds that exhibit molecular symmetry that cannot be superimposed on each other, which are also called as mirror-image isomers (or enantiomers), analogous to the relationship between the right and left hands.