The dark Macromolecular organic matter of asteroid Ryugu:
research from the Hayabusa2 Organic Macromolecule Team published in “Science”
February 24, 2023 (JST)
Japan Aerospace Exploration Agency
Hiroshima University
YOKOHAMA National University
High Energy Accelerator Research Organization
Kyushu University
Hokkaido University
Tohoku University
Kyoto University
The University of Tokyo
The Japan Aerospace Exploration Agency (JAXA) is conducting the first analysis of the sample returned from asteroid Ryugu by the Hayabusa2 mission with the Hayabusa2 Initial Analysis Team, comprising of six sub-teams and two Phase-2 curation institutes. Results from the Initial Analysis Organic Macromolecule Team was published in the US journal, Science, on February 24, 2023 (JST).
Paper title:Macromolecular organic matter in samples of the asteroid (162173) Ryugu
Journal:Science
Please refer to the Appendix for an outline.
The initial analysis of the asteroid Ryugu samples
The sample from asteroid Ryugu was returned to Earth by the Hayabusa2 spacecraft on December 6, 2020. The initial description of the sample (Phase-1 curation) followed as the facility established at JAXA’s Institute of Space and Astronautical Science (ISAS). Parts of the sample were then distributed to the Hayabusa2 Initial Analysis Team, which consists of six sub-teams and two Phase-2 curation institutes. This division into specialized sub-teams is designed to achieve the scientific objectives of Hayabusa2 through high-precision analysis of different aspects of the sample. Data from the Phase-2 curation institutes’ analysis of the grain characteristics will be included in the JAXA Hayabusa2 grain catalogue for future analysis proposals.
[Appendix]
Macromolecular organic matter in samples of the asteroid (162173) Ryugu
Key points
●
The chemical, isotopic and morphological compositions of the insoluble residues were almost consistent with those of the intact Ryugu grains, indicating that the dark organic macromolecules account for a major portion of Ryugu’s organic matter (Note 1).
●
The presence of macromolecular organic matter associated with phyllosilicates and carbonates in the Ryugu sample implies that much of the organic material was altered by low temperature aqueous processing on Ryugu’s parent body (the larger asteroid from which Ryugu is thought to be a fragment). Macromolecular organic matter in the Ryugu samples is similar that that in primitive carbonaceous chondrites, while Ryugu’s organic matter showed more diversity in composition than that in meteorites. The result provides further evidence that the organics were modified by variable degrees of aqueous alteration on Ryugu’s parent body.
●
No graphite-like material was found, which indicates that the Ryugu organic matter was not subjected to heating events on the parent body, such as long duration radiogenic thermal metamorphism and short-duration heating.
●
The observed enrichments of deuterium (D) and/or nitrogen-15(15N) indicate that at least some of the organic matter in Ryugu samples was formed in low-temperature environments below -200℃ such as the interstellar medium or presolar nebula.
●
Similarities and differences were found between the organic matter in the C-type asteroid Ryugu, and that observed in D-type asteroids and comets. This is believed to be the result of changes in the chemical reactions in each parent body, after the common source material in the solar nebula was incorporated into planetesimals (later minor bodies).
●
Dark, macromolecular organic matter in carbonaceous asteroids, which is not related to the “present” bio-related molecules, could have contributed to the organic inventory available for the formation of habitable planetary environments.
1. Overview
Hayabusa2 Initial Analysis Organic Macromolecule team led by Professor Yabuta Hikaru (Hiroshima University) measured chemical, isotopic, and morphological compositions of macromolecular organic matter in samples of the asteroid Ryugu (37 grains ranging from 200 to 900 μm in size), which were returned by the spacecraft Hayabusa2. Their coordinated analysis by various microscopic techniques showed that the chemical compositions of macromolecular organic matter in the Ryugu sample are similar to those in primitive carbonaceous CI (Ivuna-type) and CM (Mighei-type) chondritic meteorites. The morphology of the organic matter includes nanoglobules and diffuse carbon associated with phyllosilicate and carbonate minerals, which is the evidence of the evolution of organic matter during reaction with liquid water on Ryugu’s parent body (the larger asteroid from which Ryugu is thought to be a fragment). No graphite-like material was found, which indicates that the Ryugu organic matter was not subjected to heating events on the parent body, such as long duration radiogenic thermal metamorphism and short-duration heating.
A number of carbonaceous grains in the Ryugu samples showed extreme deuterium (D) and/or nitrogen-15(15N) enrichments or depletions. These isotopic features are not observed from terrestrial organic matter and indicate an origin in extreme cold environment (i.e. below negative 200 degrees Celsius). The results indicate that the organic matter in Ryugu samples is extraterrestrial origin and at least some of the organic matter were derived from the interstellar medium or presolar nebula.
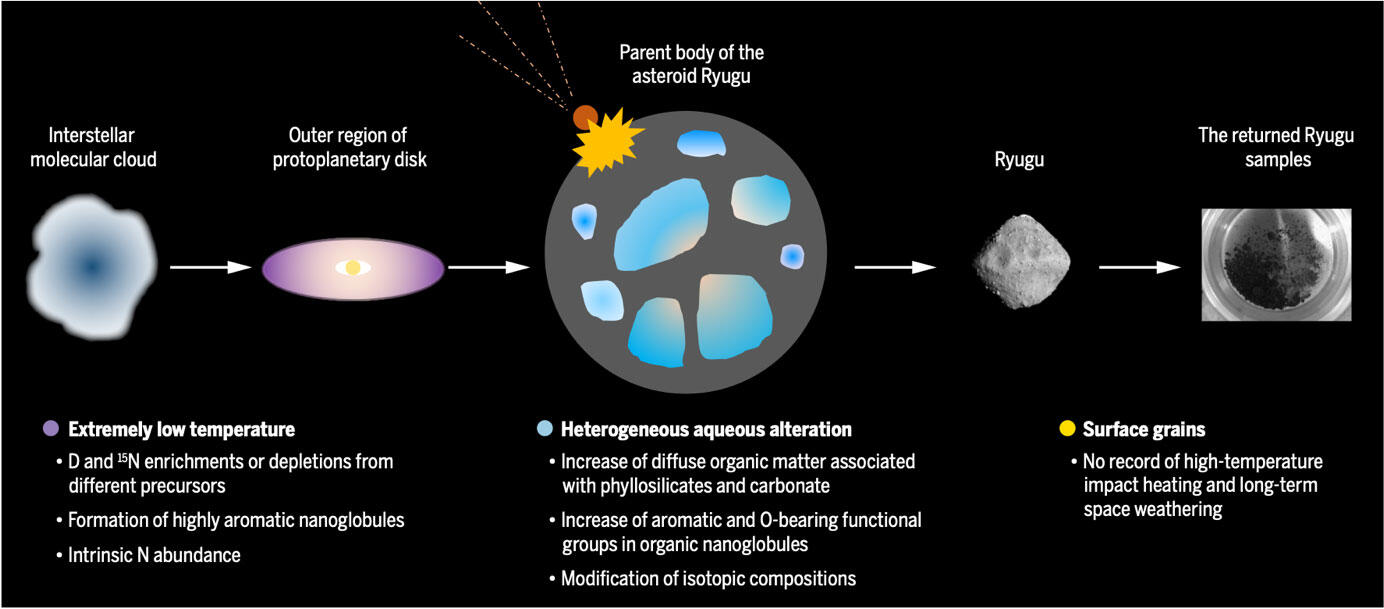
© Yabuta et al. 2023
Figure 1
Formation and evolution of macromolecular organic matter during the evolution of asteroid Ryugu
The Organic Macromolecule team examined intact Ryugu grains and insoluble carbonaceous residues isolated by acid treatment of the Ryugu samples. The measurements showed that the insoluble residues recovered in high yield was dark-colored, organic solids. The chemical, isotopic and morphological compositions of the insoluble residues were almost consistent with those of the intact Ryugu grains, indicating that the dark organic macromolecules account for a major portion of Ryugu’s organic matter. This could explain the low albedo of the asteroid’s surface.
This study has first proved the direct link between macromolecular organic matter in the carbonaceous asteroid and that in primitive carbonaceous chondrites. More specifically, organic matter in Ryugu samples showed more diversity in chemical, isotopic, and morphological compositions than that in meteorites. The result provides further evidence that the organic matter formed in interstellar cloud and solar nebula were modified by variable levels aqueous alteration on Ryugu’s parent body (Figure 1). There is no obvious difference in organic compositions between Ryugu grains collected at the first and second touchdown sites. This indicates that the organic matter formed during (or before) the Solar System’s formation is preserved in the surface samples of Ryugu and escaped solar wind, impacts and heating by sunlight on the asteroid surface.
2. Text
Organic compounds in asteroids and comets are produced during (or before) the Solar System’s formation about 4.6 billion years ago, and they have been thought of as building blocks for the planets and Earth-life. However, most of the samples used for the studies on extraterrestrial organic matter have been limited to meteorites and cosmic dusts. Organic matter has not been found from the S-type (stony) asteroid Itokawa particles returned by the first Hayabusa mission. Thus, there had been no study on the distributions of organic compounds in asteroids.
To understand the formation and evolution of organics in the C-type (carbonaceous) asteroid Ryugu in the early history of the Solar System, the Hayabusa2 Initial Analysis Organic Macromolecule team, which consisted of world experts in organic cosmochemistry, investigated the macromolecular organic matter in Ryugu samples, measuring its elemental, isotopic, and functional group compositions along with its small-scale structures and morphologies.
Analytical methods used included micro-Fourier transform infrared (FTIR) spectroscopy, micro-Raman spectroscopy, synchrotron-based scanning transmission X-ray microscopy (STXM), X-ray absorption near edge structure (XANES), scanning transmission electron microscopy (STEM) coupled with electron energy loss spectroscopy (EELS) and energy dispersive X-ray spectroscopy (EDS), atomic force microscope based infrared (AFM-IR) spectroscopy, and nanometer-scale secondary ion mass spectrometry (NanoSIMS). Intact Ryugu grains and insoluble carbonaceous residues isolated by acid treatment of the Ryugu samples were examined, respectively.
2-1. Significance of acid treatment
In-situ analysis of Ryugu intact samples (non-destructive) has an advantage of investigating chemical and isotopic heterogeneities of organic matter without modification of the samples. On the other hand, the non-destructive analysis is not good at measurement of bulk compositions, which characterize asteroid Ryugu. Also, in-situ analysis of H, O, and S of organic matter mixed with minerals are sometimes challenging due to interference by H, O, and S from the minerals themselves (e.g. phyllosilicates, sulfides). Therefore, to analyze highly refined macromolecular organic matter, in this study, insoluble carbonaceous residues were purified and isolated from Ryugu samples through demineralization by continuous acid (HF/HCl) treatment over one month period. The measurements showed that the insoluble residues recovered in high yield and in high purity was dark-colored organic solids (Figure 2). The chemical, isotopic and morphological compositions of the insoluble residues were almost consistent with those of the intact Ryugu grains (Figures 3, 6, and 9), indicating that the dark organic macromolecules account for a major portion of Ryugu’s organic matter.
Slight differences between the Ryugu intact samples and insoluble carbonaceous residues from the Ryugu samples (Figures 3, 5, and 9) appears to be due to i) chemical heterogeneities, ii) possible contributions of minerals and soluble organics in the intact samples, iii) possible modification of organic matter associated with phyllosilicates by HF attack on phyllosilicates, or iv) partial hydrolysis of macromolecular organic matter by acid treatment. Moreover, this study was even able to reveal chemical and isotopic heterogeneities from the carbonaceous residues from Ryugu samples.
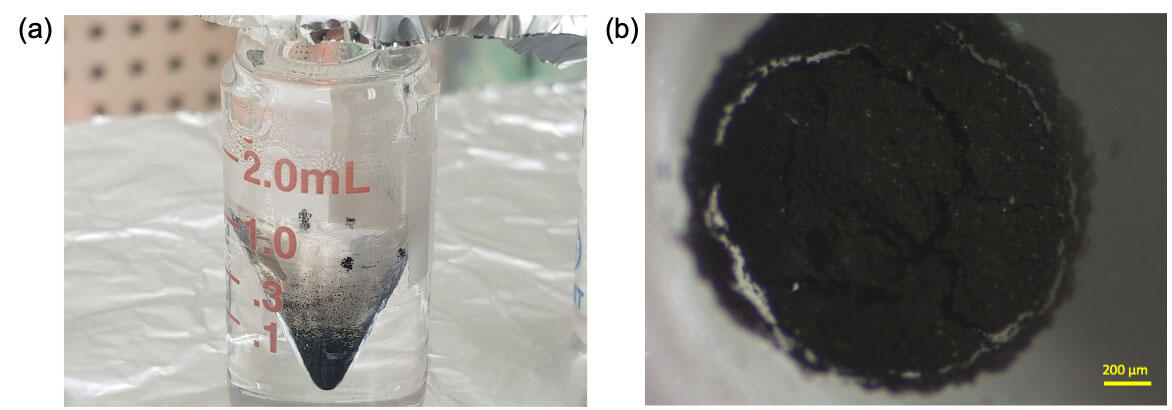
© Yabuta et al. 2023
Figure 2
Images of insoluble carbonaceous residues isolated from the intact Ryugu aggregates (A0106) by HF/HCl treatment. (A) The Ryugu carbonaceous residue in a mini glass vial. (B) An overhead image of the Ryugu carbonaceous residue aliquots transferred in another mini vial.
2-2. Relationship between organic matter in the carbonaceous asteroid and that in primitive carbonaceous chondrites
Micro-FTIR, micro-Raman, and STXM-XANES measurements of Ryugu intact samples (20 grains in total) showed that the Ryugu organic matter consists of aromatic carbons, aliphatic carbons, ketones, and carboxyls. The functional group compositions are consistent with those of insoluble organic matter (IOM) from primitive carbonaceous CI (Ivuna-type) and CM (Mighei-type) chondritic meteorites (Figures 3, 4, and 6). On the other hand, no graphite-like ordered structure was found in Ryugu’s organic matter. In general, graphite-like matter is formed by heating of organic matter at high temperature (carbonization). Spectral difference between organic matter in Ryugu samples and that in thermally metamorphosed carbonaceous chondrites (Figure 4,) indicates that the Ryugu organic matter was not subjected to heating events on the parent body at higher temperature than 200℃, such as long duration radiogenic thermal metamorphism and short-duration heating.
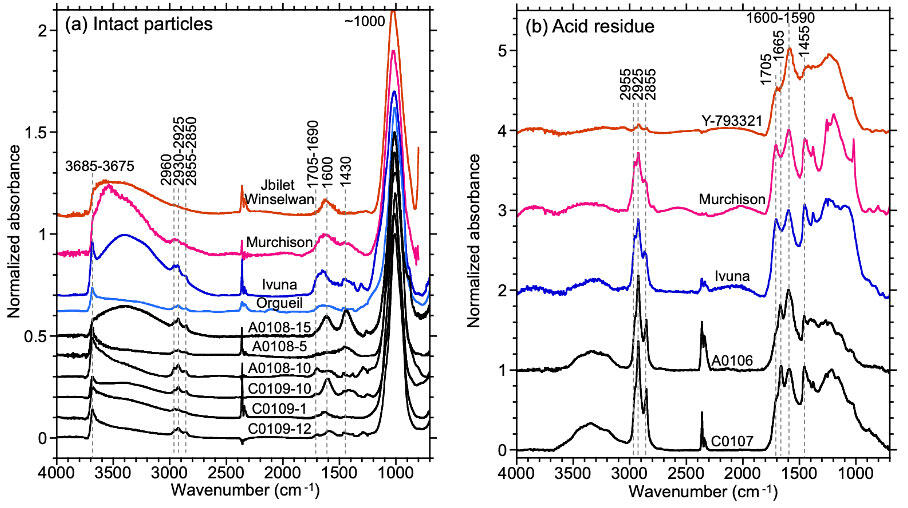
© Yabuta et al. 2023
Figure 3
Micro-FTIR spectra of Ryugu samples compared with chondrites. (a) Infrared transmission spectra of Ryugu intact grains and chondrites. (b) Same as (A), but for insoluble carbonaceous residues from the Ryugu samples compared with those from carbonaceous chondrites. Absorption bands derived from aliphatic C-H (2960 cm -1, 2930-2925 cm-1, 2855-2850 cm-1, 1460 cm-1, 1380 cm-1), C=O (1705-1690 cm-1), and aromatic C=C(~1600 cm-1) are observed.
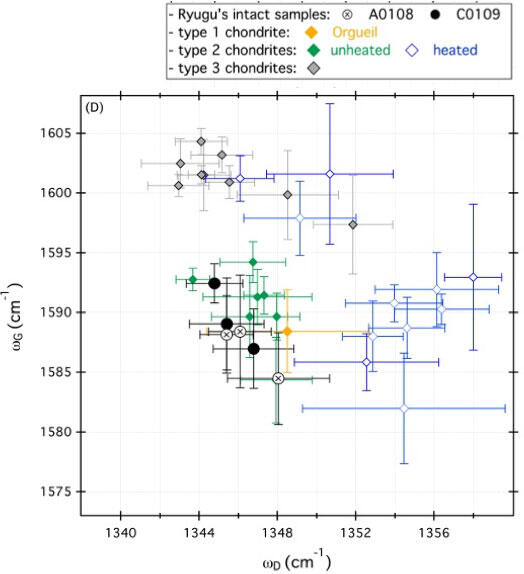
© Yabuta et al. 2023
Figure 4
Comparison of Raman spectral parameters [peak positions (wG as a function of wD) of the D- and G-bands, which are derived from polyaromatic structures] between Ryugu intact samples and chondrites.
NanoSIMS measurements of the Ryugu intact samples (4 grains) showed that the bulk hydrogen and nitrogen isotopic ratios(Note 2)of the Ryugu grains are between the bulk values of CI chondrites and the insoluble organic matter (IOM) in CI chondrites (Figure 5). These results indicate that organic matter in the Ryugu samples is chemically and isotopically similar to those in chemically primitive carbonaceous chondrites. The conclusion has a consensus with those obtained from elemental compositions, mineralogy, and noble gas isotopic compositions of the Ryugu samples.
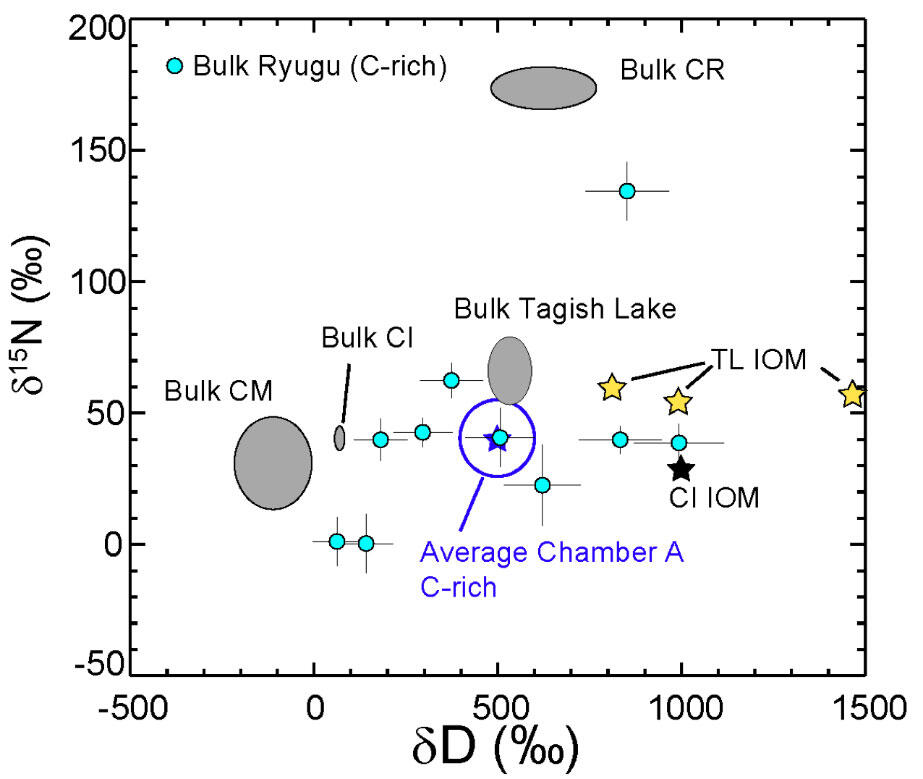
© Yabuta et al. 2023
Figure 5
The δ15N as a function of δD for bulk carbon-rich material in the intact Ryugu grains in comparison with primitive carbonaceous chondrites.
2-3. Interaction among organics, minerals, and water on Ryugu’s parent body observed by nanometer-scale observations
Several microscopic techniques with high spatial resolution, such as STXM-XANES, STEM-EELS-EDS, were applied to Ryugu intact samples (12 grains in total). The functional group distributions of the Ryugu organic matter varied on submicrometer scales in ways that relate to the morphologies: nanoparticulate and/or nanoglobular regions were aromatic-rich, whereas organic matter associated with Mg-rich phyllosilicate matrix and carbonates was meteoritic IOM-like or occurred as diffuse carbon (Figures 6, 7, and 8). Diffuse carbon sometimes contained molecular carbonate.
Phyllosilicates and carbonates likely formed through aqueous alteration. The presence of organic matter associated with these minerals implies that much of the organic material was altered by low temperature aqueous processing on Ryugu’s parent body. Progressive aqueous alteration causes:
i) an increase in the amount of diffuse organic matter associated with phyllosilicates through hydrolysis of primordial macromolecular organic matter or intercalation and oxidation of soluble molecules,
ii) an increase in the proportion of aromatic and oxygen-bearing functional groups in discrete organic particles and nanoglobules through aromatization and oxidation of macromolecular organic matter, and
iii) an increase in macromolecular diversity.
The observation results were similar between Ryugu intact grains and insoluble carbonaceous residues from Ryugu samples, but molecular carbonate was not observed from insoluble carbonaceous residues. The difference implies that organic matter containing molecular carbonate is susceptible to acid.
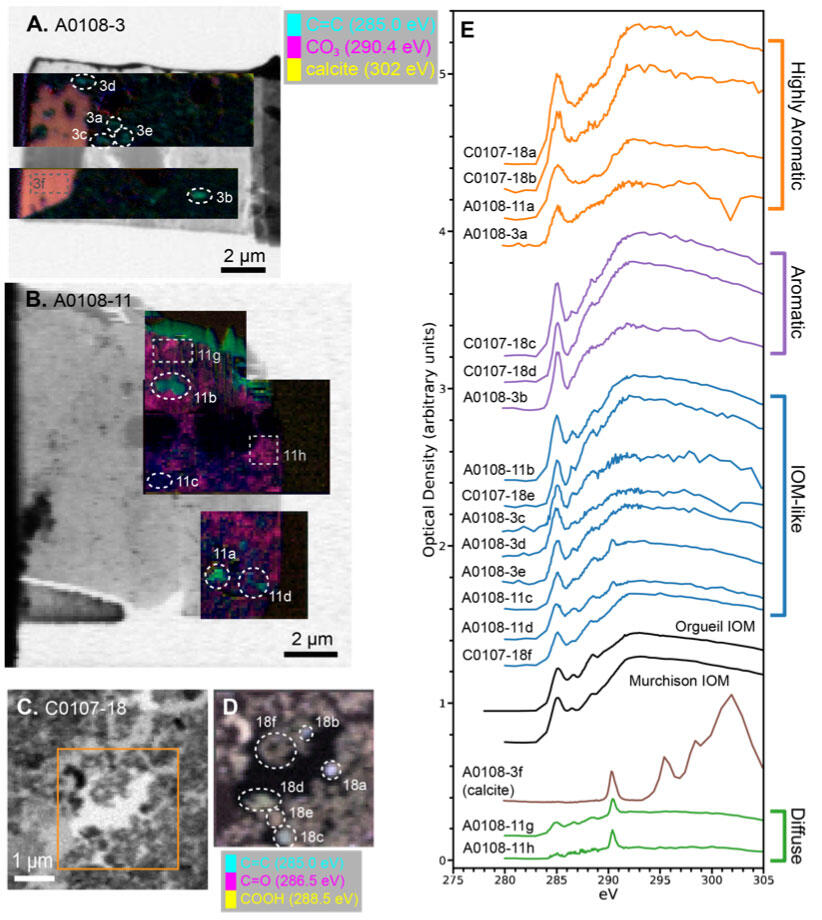
© Yabuta et al. 2023
STXM elemental maps and carbon-XANES spectra of Ryugu samples.
Figure 6
(A and B) STXM images of ultrathin sections extracted from the Ryugu grains. In both panels, color overlays on both sections are falsecolor maps of x-ray absorptions due to individual functional groups (see the figure legend). (C) STXM image of insoluble carbonaceous residue from Ryugu sample. The orange box shows the region in (D). (D) X-ray absorption map of individual functional groups (see the figure legend). (E) Carbon-XANES spectra for carbonaceous grains and matrix regions identified in (A), (B), and (D). Carbon-XANES spectra show three major peaks, resulting from aromatic carbon (C=C, 285 eV), aromatic ketone (C=C-C=O, 286.7 eV), and carboxyl (COOH, 288.5 eV) functional groups. Some spectra contain an additional peak at 290.4 eV, which corresponds to carbonate groups. IOM from the meteorites is shown for comparison.
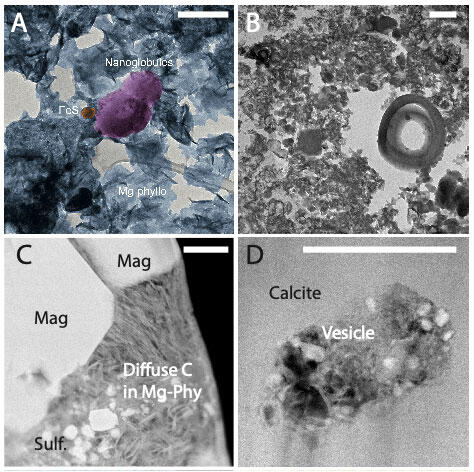
© Yabuta et al. 2023
Scanning transmission electron microscope (STEM) analysis of Ryugu samples.
Figure 7
All scale bars are 500 nm. (A) TEM image of the ultrathin section of Ryugu sample, indicating nanoglobules (magenta), Mg-phyllosilicates (blue), and FeS (orange). (B) TEM image of insoluble organic residue from Ryugu sample, showing hollow and solid nanoglobules and fluffy materials. (C) TEM image of Ryugu sample (region 11h in Fig. 6B) showing magnetite grains (Mag) adjacent to carbon-bearing Mg-rich phyllosilicates (Mg-phy) with Fe and Ni sulfide nanoparticles (Sulf). (D) TEM image of a vesicle in the large calcite grain from Ryugu sample (region 3a in Fig. 6A).
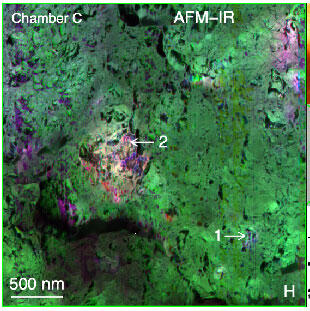
© Yabuta et al. 2023
AFM-IR analysis of intact Ryugu grains.
Figure 8
Organic matter in the Ryugu grain appears as red-purple inclusions standing out from the dominant phyllosilicate signal. Colors indicate the C=O (1720 cm-1, red), C=C (1600 cm-1, blue) and Si-O (1020 cm-1, green) peaks.
2-4. The site where organic matter first formed was a low-temperature environment prior to the formation of Ryugu’s parent body
Extreme deuterium (D) and/or nitrogen-15(15N) enrichments or depletions were detected both from Ryugu intact samples and insoluble organic residues by nanoSIMS measurements. These isotopic features are not observed from terrestrial organic matter and indicate an origin in an extreme cold environment (i.e., below negative 200 degrees Celsius). The results indicate that the organic matter in Ryugu samples is extraterrestrial origin and at least some of the organic matter it is derived from the interstellar medium or presolar nebula.
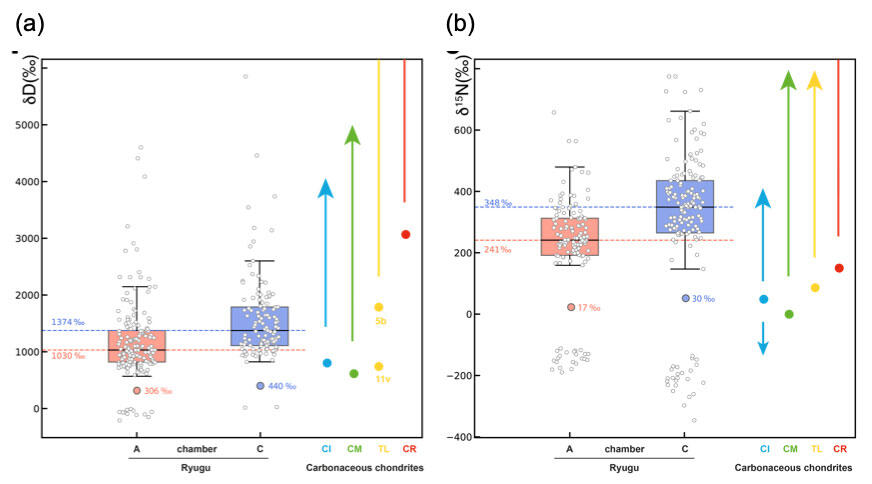
© Yabuta et al. 2023
Figure 9
NanoSIMS analysis of insoluble carbonaceous residues from Ryugu grains. Box plot diagrams show the distributions of hydrogen (I) and nitrogen (J) isotope ratios in the insoluble carbonaceous residues from Ryugu samples. Open grey circles show hot-spots (or cold-spots), micron-sized areas with isotope ratios much higher (or lower) than the average composition. Large orange and blue circles indicate the bulk average value for each residue. Horizontal dashed lines indicate the mode of each distribution of hotspots. Data for the insoluble organic matter from chondrites are shown for comparison. Filled circles are bulk values.
2-5. Relationship between C-type asteroid Ryugu, D-type asteroid, and comets
The macromolecular organic matter of the C-type asteroid Ryugu was compared with other primitive small bodies in the early Solar System. The ungrouped Tagish Lake C2 carbonaceous chondrite is thought to be related to the dark (D-type) asteroids, which are located in the outer main asteroid belt and among Jupiter’s trojan asteroids. Tagish Lake contains organic and mineralogical variations between different lithologies that reflect variable degrees of alteration on the same parent body. The distributions of D-enrichments in the Ryugu sample were within the range of CI, CM, and Tagish Lake chondrites, respectively. It is noted that the distributions of the D-enrichments within the Ryugu sample were even within the most altered lithology (11v) and the least altered lithology (5b) of Tagish Lake chondrites, respectively. On the other hand, the δD distributions of the Ryugu IOM are shifted lower than those in less altered CR chondrites, and interplanetary dust particles (IDPs) and Antarctic micrometeorites (AMMs) which are derived in comets. Consistency in the distributions of D- (and 15N-) enrichments of IOM between Ryugu and aqueously altered primitive carbonaceous chondrites indicate that Ryugu organic matter likely resulted from heterogeneous aqueous processing that occurred on C- and D-type asteroids from the common primordial materials formed at an earlier stage of the solar nebula.
The atomic ratio of nitrogen to carbon of insoluble carbonaceous residues from Ryugu samples is similar to those of CI and CM chondrites. In contrast, N/C of Tagish Lake IOM is slightly higher than that of Ryugu. In addition, N/C of 81P/Wild2 cometary dusts, IDPs and AMMs are significantly higher than that of Ryugu. Laboratory experiment shows that the N/C ratios in meteoritic IOM are not substantially modified by reaction with water, and thus the N abundance in Ryugu organic matter could have been determined in interstellar molecular cloud and solar nebula. The observed similarities and differences among C-type asteroid Ryugu, D-type asteroid, and comets imply a continuity of source material in the solar nebula.
2-6. Dark-colored organic matter has a potential to contribute to the formation of habitable planets
It has been thought that organic compounds in asteroids and comets might have been delivered to the early Earth as life’s building blocks. To date, only biologically-related molecules, such as amino acids, sugar, and nucleobases in meteorites, have been the focus of candidates for life’s building blocks. However, this study showed that the dark, coal-like organic macromolecules accounted for a major portion of organic matter in the carbonaceous asteroid Ryugu. Complex aromatic macromolecules are not related to the “present” bio-related molecules, but would have been delivered to the early Earth and may have formed the first functional organic material, through further chemical reactions on the early Earth. It has been shown that the acid-insoluble macromolecular organic matter in CM chondrite released a number of (soluble) organic molecules and reducing volatiles by hydrous pyrolysis experiment. Thus, this study leads a new hypothesis that macromolecular organic matter in carbonaceous asteroids could have contributed to the organic inventory available for the formation of habitable planetary environments.
3. Paper information
Journal:
Science
Paper title:
Macromolecular organic matter in samples of the asteroid (162173) Ryugu
Authors:Yabuta1, G. D. Cody2, C. Engrand3, Y. Kebukawa4, B. D. Gregorio5, L. Bonal6, L. Remusat7, R. Stroud5, E. Quirico6, L. Nittler2, M. Hashiguchi8, M. Komatsu9, 10, T. Okumura11, J. Mathurin12, E. Dartois13, J. Duprat7, Y. Takahashi11, 14, Y. Takeichi14, D. Kilcoyne15¶, S. Yamashita14, A. Dazzi12, A. Deniset-Besseau12, S. Sandford16, Z. Martins17, Y. Tamenori18, T. Ohigashi19, H. Suga18, D. Wakabayashi14, M. Verdier-Paoletti7, S. Mostefaoui7, G. Montagnac20, J. Barosch2, K. Kamide1, M. Shigenaka1, L. Bejach3, M. Matsumoto21, Y. Enokido21, T. Noguchi22, H. Yurimoto23, T. Nakamura21, R. Okazaki24, H. Naraoka24, K. Sakamoto25, H. C. Connolly Jr.26, D. S. Lauretta27, M. Abe25, 28, T. Okada25, 29, T. Yada25, M. Nishimura25, K. Yogata25, A. Nakato25, M. Yoshitake25, A. Iwamae30, S. Furuya11, K. Hatakeda30, A. Miyazaki25, H. Soejima30, Y. Hitomi30, K. Kumagai30, T. Usui25, T. Hayashi25, D. Yamamoto25, R. Fukai25, S. Sugita11, 31, K. Kitazato32, N. Hirata32, R. Honda33, T. Morota11, E. Tatsumi34, N. Sakatani35, N. Namiki28, 36, K. Matsumoto28, 36, R. Noguchi37, K. Wada31, H. Senshu31, K. Ogawa38, Y. Yokota25, Y. Ishihara38, Y. Shimaki25, M. Yamada31, C. Honda32, T. Michikami39, M. Matsuoka40, N. Hirata41, M. Arakawa41, C. Okamoto41¶, M. Ishiguro42, R. Jaumann43, J.-P. Bibring44, M. Grott45, S. Schröder46, K. Otto1, 45, C. Pilorget44, 47, N. Schmitz45, J. Biele48, T.-M. Ho49, A. Moussi-Soffys50, A. Miura25, H. Noda28, 36, T. Yamada25, K. Yoshihara25, K. Kawahara25, H. Ikeda25, Y. Yamamoto25, K. Shirai41, S. Kikuchi31, 36, N. Ogawa25, H. Takeuchi25, 28, G. Ono51, Y. Mimasu25, K. Yoshikawa51, Y. Takei25, A. Fujii25, Y. Iijima25¶, S. Nakazawa25, S. Hosoda25, T. Iwata25, 28, M. Hayakawa25, H. Sawada38, H. Yano25, 28, R. Tsukizaki25, M. Ozaki25, 28, F. Terui52, S. Tanaka25, 28, M. Fujimoto25, M. Yoshikawa25, 28, T. Saiki25, S. Tachibana11, 25, S. Watanabe8, Y. Tsuda25
1Department of Earth and Planetary Systems Science, Hiroshima University, Hiroshima 739-8526, Japan.
2Earth and Planets Laboratory, Carnegie Institution for Science, Washington, DC 20015, USA.
3Laboratoire de Physique des 2 Infinis Irène Joliot-Curie, Université Paris-Saclay, Centre National de la Recherche Scientifique, 91405 Orsay, France.
4Faculty of Engineering, Yokohama National University, Yokohama 240-8501, Japan.
5Materials Science and Technology Division, US Naval Research Laboratory, Washington, DC 20375, USA.
6Institut de Planétologie et d'Astrophysique, Université Grenoble Alpes, 38000 Grenoble, France.
7Institut de Mineralogie, Physique des Materiaux et Cosmochimie, Museum National d'Histoire Naturelle, Centre National de la Recherche Scientifique, Sorbonne Université, 75231 Paris, France.
8Graduate School of Environmental Studies, Nagoya University, Nagoya 464-8601, Japan.
9Center for University-Wide Education, Saitama Prefectural University, Saitama 343-8540, Japan.
10Department of Earth Sciences, Waseda University, Tokyo 169-8050, Japan.
11Department of Earth and Planetary Science, The University of Tokyo, Tokyo 113-0033, Japan.
12Institut Chimie Physique, Université Paris-Saclay, Centre National de la Recherche Scientifique, 91405 Orsay, France.
13Institut des Sciences Moléculaires d'Orsay, Université Paris-Saclay, Centre National de la Recherche Scientifique, 91405 Orsay, France.
14Institute of Materials Structure Science, High Energy Accelerator Research Organization, Tsukuba 305-0801, Japan.
15Advanced Light Source, Lawrence Berkeley National Laboratory, Berkeley 94720-8229, USA.
16NASA Ames Research Center, Moffett Field 94035-1000, USA.
17Centro de Química Estrutural, Institute of Molecular Sciences and Department of Chemical Engineering, Instituto Superior Técnico, Universidade de Lisboa, Lisboa 1049-001, Portugal.
18Japan Synchrotron Radiation Research Institute, Hyogo 679-5198, Japan.
19Institute for Molecular Science, UVSOR Synchrotron Facility,Okazaki 444-8585, Japan.
20École normale supérieure de Lyon, University Lyon 1, 69342 Lyon, France.
21Department of Earth Science, Tohoku University, Sendai 980-8578, Japan.
22Department of Earth and Planetary Sciences, Kyoto University, Kyoto 606-8502, Japan.
23Department of Earth and Planetary Sciences, Hokkaido University, Sapporo 060-0810, Japan.
24Department of Earth and Planetary Sciences, Kyushu University, Fukuoka 819-0395, Japan.
25Institute of Space and Astronautical Science, Japan Aerospace Exploration Agency (JAXA), Sagamihara 252-5210, Japan.
26Department of Geology, School of Earth and Environment, Rowan University, Glassboro 08028, USA.
27Lunar and Planetary Laboratory, University of Arizona, Tucson 85721, USA.
28School of Physical Sciences, The Graduate University for Advanced Studies, Hayama 240-0193, Japan.
29Department of Chemistry, The University of Tokyo, Tokyo 113-0033, Japan.
30Marine Works Japan Ltd., Yokosuka 237-0063, Japan.
31Planetary Exploration Research Center, Chiba Institute of Technology, Narashino 275-0016, Japan.
32Aizu Research Cluster for Space Science, University of Aizu, Aizu-Wakamatsu 965-8580, Japan.
33Center of Data Science, Ehime University, Matsuyama 790-8577, Japan.
34Instituto de Astrofísica de Canarias, University of La Laguna, Tenerife E-38205, Spain.
35Department of Physics, Rikkyo University, Tokyo 171-8501, Japan.
36Research of Interior Structure and Evolution of Solar System Bodies, National Astronomical Observatory of Japan, Mitaka 181-8588, Japan.
37Faculty of Science, Niigata University, Niigata 950-2181, Japan.
38JAXA Space Exploration Center, Japan Aerospace Exploration Agency (JAXA), Sagamihara 252-5210, Japan.
39Faculty of Engineering, Kindai University, Higashi-Hiroshima 739-2116, Japan.
40Geological Survey of Japan, National Institute of Advanced Industrial Science and Technology, Tsukuba 305-8567, Japan.
41Department of Planetology, Kobe University, Kobe 657-8501, Japan.
42Department of Physics and Astronomy, Seoul National University, Seoul 08826, Republic of Korea.
43Freie Universität Berlin, Institute of Geological Sciences, Planetary Sciences and Remote Sensing, Berlin 12249, Germany.
44Institut d'Astrophysique Spatiale, Université Paris-Saclay, Centre National de la Recherche Scientifique, 91405 Orsay, France.
45Institute of Planetary Research, German Aerospace Center, Berlin 12489, Germany.
46Department of Computer Science, Electrical and Space Engineering, Luleå University of Technology, 98128 Kiruna, Sweden.
47Institut Universitaire de France, 75005 Paris, France.
48Microgravity User Support Centre, German Aerospace Center, Cologne 53147, Germany.
49Institute of Space Systems, German Aerospace Center, Bremen 28359, Germany.
50Centre National d’Études Spatiales, 31401 Toulouse, France.
51Research and Development Directorate, JAXA, Sagamihara 252-5210, Japan.
52Department of Mechanical Engineering, Kanagawa Institute of Technology, Atsugi 243-0292, Japan.
¶Deceased.
DOI No.:10.1126/science.abn9057
4. Notes
(Note 1) Model structure of macromolecular organic matter in carbonaceous chondrite meteorite (CM group), which is similar to that in Ryugu, is shown below (Glavin et al. 2018). Based on a number of studies, it has been suggested that the macromolecular organic matter has a complex, disordered structure containing a molecular network of one to six ring-aromatic units crosslinking with short-branched aliphatic chains and various oxygen functional groups, such as carboxyl, ketone, and ether groups. Its elemental composition is expressed as C100H75O17N4S3 (Alexander et al. 2007). Because of its complex structure, the whole molecular compositions are still unknown.
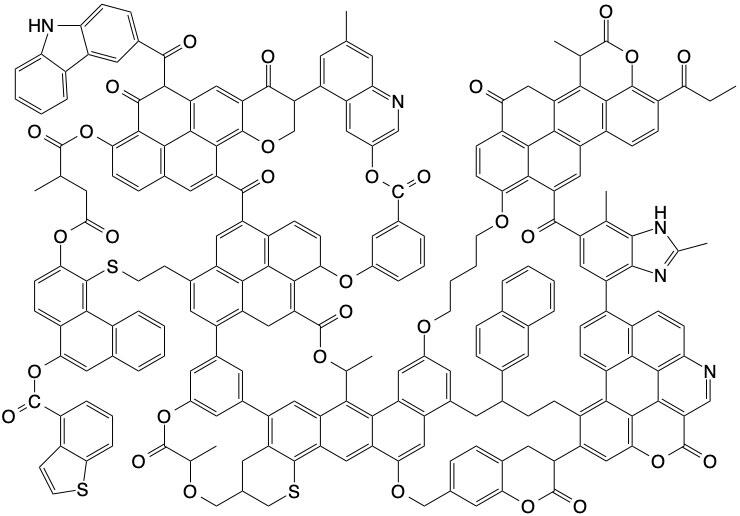
©Glavin et al. 2018
(Note 2) Isotopic ratios are expressed in delta units, following the relation:
δR (‰)= [(Rsample/Rstandard) − 1] x 1000
where R sample is the sample isotopic ratio and R standard is the ratio of a terrestrial standard.